What is ultrafast laser? Ultrafast lasers, also known as ultrashort pulse lasers, have emerged as a revolutionary technology in material processing and precision applications. In industrial applications, lasers are typically categorized into four main types: continuous wave, quasi-continuous, short pulses, and ultrashort pulses. Pico and femtosecond laser features extremely short pulse widths, typically in the femtosecond (10⁻¹⁵ seconds) or picosecond (10⁻¹² seconds) range. This ultrashort pulse duration imparts unique properties to ultrafast lasers, making them suitable for a wide range of applications.
The successful development of ultrashort pulse lasers can be attributed to significant breakthroughs in mode-locking technology. This technique cleverly introduces a fixed phase relationship within the laser cavity, causing interference between different modes, which results in the generation of a series of laser pulses. Through this method, the output pulse width of the laser is dramatically shortened to the picosecond or femtosecond range, placing it within the domain of ultrashort pulses. These laser pulses, commonly referred to as ultrafast lasers, include industrial laser types such as picosecond lasers, femtosecond lasers, and even the cutting-edge attosecond lasers used in scientific research.
What is Ultrafast Laser Processing?
Ultrafast laser processing refers to the use of extremely short laser pulses, typically in the femtosecond (fs) to picosecond (ps) range, to perform precise material processing tasks. These short pulses enable highly localized energy delivery, allowing for intricate and controlled interactions with the material being processed, with minimal thermal effects. As a result, ultrafast laser machine is used for applications like micro-machining, surface structuring, engraving, and even medical procedures, providing high precision and minimal damage to the surrounding material.
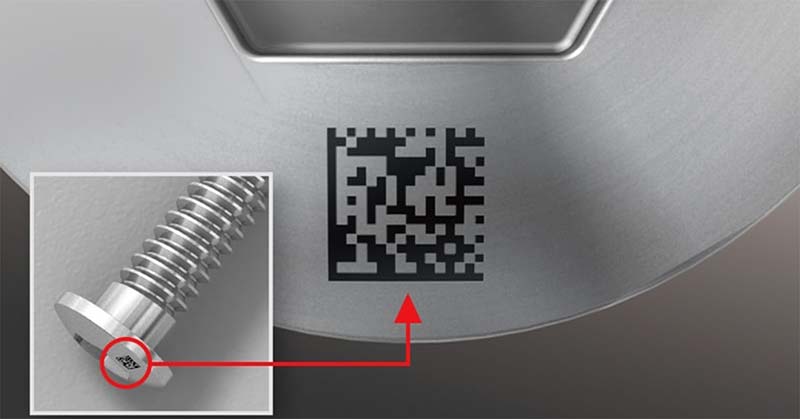
What are the advantages of ultrashort pulse lasers in material processing compared to short-pulse lasers?
Traditional lasers work by accumulating thermal energy, which causes the material in the target area to melt or even vaporize. This process can lead to defects such as debris and micro cracks, and the longer the laser duration, the greater the damage to the material. In contrast, ultrashort pulse lasers interact with the material for an extremely short time, with single-pulse energy strong enough to ionize any material, enabling non-thermal, cold processing. This results in processing advantages such as ultra-fine precision and minimal damage, which are unattainable with longer pulse lasers.
Additionally, ultrafast lasers offer a broader range of material compatibility, making them suitable for sensitive surfaces such as metals, TBC coatings, glass, plastics, and more.
With extremely high processing precision, ultrafast lasers utilize the strong-field effects of the laser to directly break molecular bonds, enabling ultra-precise, cold machining of materials.
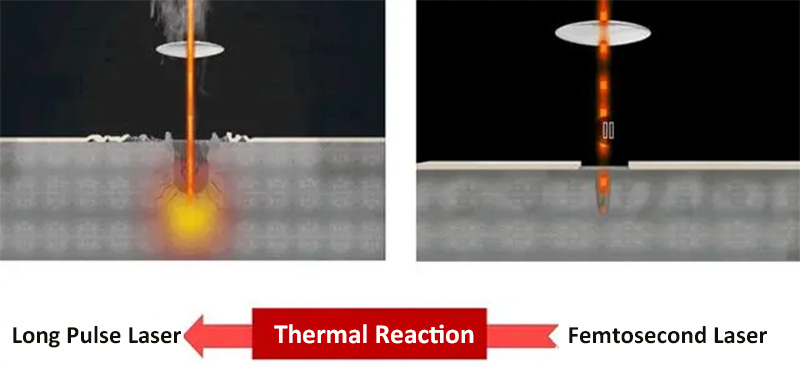
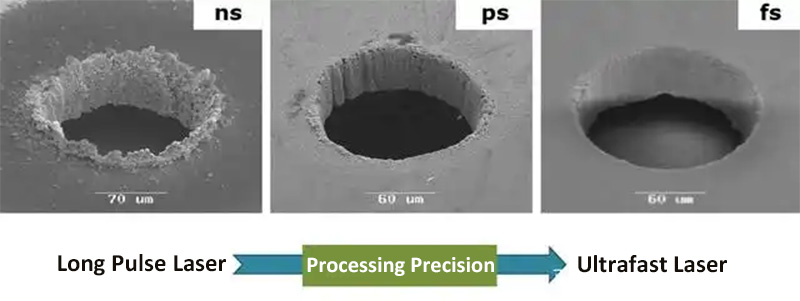
The two core elements of an ultrafast laser are: generating highly stable ultrashort pulses and achieving high pulse energy. By using mode-locking technology, we can obtain the desired ultrashort pulses, while the CPA (chirped pulse amplification) technique further amplifies the pulse energy. In this process, key components such as the oscillator, stretcher, amplifier, and compressor play a crucial role.
(1) Laser Mode-Locking Technology
Mode-locking is one of the key technologies for generating ultrashort pulses in lasers. Similar to Q-switching, mode-locking also modulates the laser cavity to split continuous wave (CW) light into pulses. However, it is important to note that although the two phenomena are related, the underlying physical principles are fundamentally different.
Mode-locking technology can generally be divided into active mode-locking and passive mode-locking. In practical applications, besides active phase modulation, loss modulation is a more commonly used mode-locking method, with the saturated absorption effect widely applied to achieve mode-locking. The saturated absorption effect is characterized by a gradual decrease in the material’s absorption of light as the light intensity increases. When the light intensity reaches a certain threshold, the saturable absorber (SA) stops absorbing light and becomes transparent.
Currently, industrial-grade fiber mode-locked seed sources often use SESAM-based mode-locking combined with a linear cavity design. By selecting appropriate output chirped gratings and SESAM parameters, precise control of picosecond or femtosecond mode-locking can be achieved.
(2) CPA Amplification Technology
On October 2, 2018, the Nobel Prize in Physics was awarded to American scientist Arthur Ashkin, French scientist Gerard Mourou, and his student Donna Strickland. They shared this prestigious honor, with half of the award going to Ashkin for his contributions to “optical tweezers and their application to biological systems,” and the other half awarded to Mourou and Strickland for their breakthrough in Chirped Pulse Amplification (CPA) technology. CPA has provided an effective means for generating high-intensity ultrashort pulse lasers.
Since its inception in the 20th century, mode-locking technology has evolved through active, passive, and Kerr-lens self-mode-locking stages, greatly advancing the generation of femtosecond-level ultrashort pulses. With the rise of titanium-sapphire femtosecond laser oscillators, a new era emerged where more integrated and stable mode-locked lasers gradually replaced earlier dye lasers, thus leading ultrashort pulse lasers into broader application fields. However, directly amplifying the ultrashort pulses output from the oscillator faces a challenge: nonlinear effects within the laser crystal can cause the formation of filaments or even damage the crystal. This not only affects the efficient amplification of energy but also harms the crystal components.
To overcome this issue, Mourou and Strickland innovatively proposed Chirped Pulse Amplification (CPA). They cleverly broadened the pulses output by the oscillator in the time domain, significantly reducing the peak power density and eliminating most nonlinear effects. In this way, the seed pulse can absorb enough energy from the pump light for optical amplification. The pulse is then compressed by a compression element to output a short pulse. This breakthrough allows the energy of a single pulse to reach levels as high as millijoules or even joules. By further focusing the ultrashort pulses, peak power densities as high as 10^19 W/cm² can be achieved at the focal point, providing a solid experimental foundation for studying the interaction mechanisms and applications of ultrafast, high-intensity lasers with matter. The birth of ultrashort pulse lasers and CPA technology marks the beginning of a new developmental stage in laser processing technology.
What Are Ultrashort Pulse Laser Applications?
Ultrafast pulse lasers, with their high peak power, can induce intense nonlinear effects. When the laser intensity reaches a certain threshold, the optical properties of the material undergo significant changes, leading to nonlinear phenomena such as multiphoton absorption, second harmonic generation, and self-focusing. These nonlinear effects open up new avenues for laser technology in fields such as material processing, optical frequency conversion, and ultrashort pulse generation.
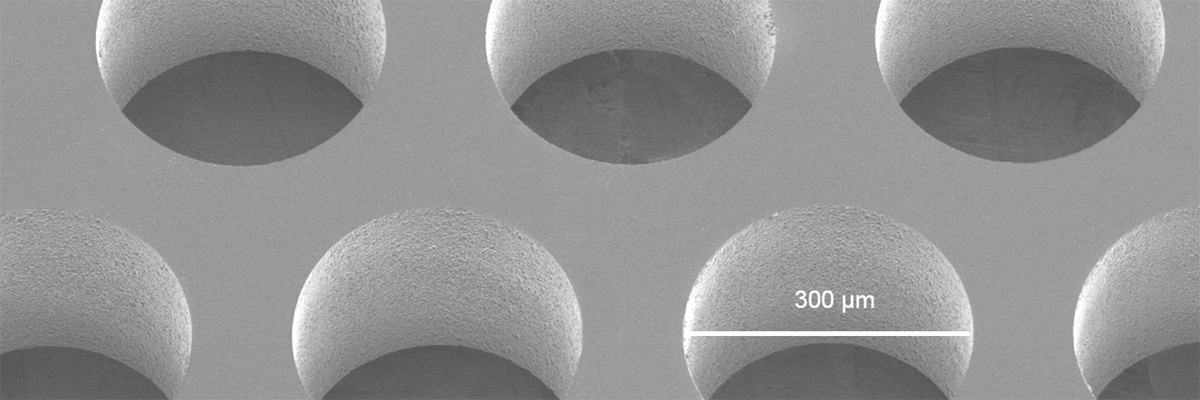
Applications of Ultrafast Lasers in Material Processing
In the field of material science, ultrafast pulse lasers can be used for high-precision micro-machining of materials. By controlling laser parameters, it is possible to achieve micron-level processing accuracy and the fabrication of complex structures. Ultrafast laser technology is widely applied in areas such as:
- Micro-hole drilling
- Microelectronics component manufacturing
- Glass cutting
- Stainless steel color marking
- Semiconductor processing
- PCB (Printed Circuit Board) and FPC (Flexible Printed Circuit) manufacturing
These applications provide strong support for precision manufacturing, allowing for high-quality, intricate, and efficient processing in industries that require fine detail and complex designs.
Ultrafast Lasers in Display Panel Cutting
By shaping the beam of an ultra-fast laser, it is possible to obtain a Bessel beam with a long depth of focus, which is precisely applied to the glass substrate of the display panel, thus enabling the cutting of arbitrary shapes. The Bessel laser cuts transparent and brittle materials in two steps: firstly, the laser cuts, and then, with the help of an external force, the material is lobed. It is worth noting that the difficulty of fragmentation rises significantly as the thickness of the material being cut increases. To meet this challenge, various strategies can be used, such as multiple cuts to compensate for the lack of depth of focus, or increasing the laser pulse energy and designing a cutting head with a longer depth of focus.
In addition, technological innovations should not be overlooked. For example, the use of burst-mode pulses can further optimize cutting results. However, for particularly thick materials or complex cutting requirements, technological enhancements alone may not be sufficient and need to be combined with innovations in laser technology to achieve higher pulse energies.

Ultrafast Laser in FPC and OLED Cutting
The polymer material of OLEDs is very sensitive to thermal influences and modern production of very small cell sizes and spacing’s has made the traditional die-cutting process incapable of meeting current production demands. At the same time, the emergence of innovative applications such as shaped and perforated screens is beyond the capabilities of traditional processes. However, ultrafast laser technology, particularly picosecond UV and femtosecond lasers, with their small heat-affected zones and curved processing flexibility, are ideal for solving these problems.
Micro-Welding Applications with Ultrafast Lasers
When working with transparent solid media such as glass, the propagation of ultrashort pulsed lasers triggers a series of complex physical phenomena. These phenomena include nonlinear absorption, melting damage, plasma formation, ablation, and filament propagation. Through the illustration, we can clearly see the various phenomena that occur when the ultrashort pulsed laser interacts with solid materials at different power densities and time scales. These phenomena provide strong support for fine processing such as micro-welding.
Due to the many advantages of ultrashort pulsed laser micro-welding technology, such as no need to insert an intermediate layer, high efficiency, high precision, no macroscopic thermal effects, as well as after welding the material to maintain the ideal mechanical and optical properties, it has become an ideal choice for the micro-welding of transparent materials such as glass. In fact, researchers have successfully used this technique to perform end-cap welding for both standard and microstructure optical fibers using 70 femtosecond, 250 kilohertz pulses.
Applications of Ultrafast Lasers in Medicine
In the medical field, ultrafast pulse lasers are used in ophthalmic surgeries, skin treatments, and other procedures, offering advantages such as minimal trauma and quick recovery. Ultrafast lasers are particularly useful in the precision cutting of minimally invasive surgical instruments, such as catheters, scalpels, and stents. The extremely high processing precision of ultrafast lasers meets the requirements for complex geometries and micron-level details. For example, the walls of cardiac stents are very thin, and traditional mechanical cutting methods can no longer meet the processing needs. In such cases, femtosecond laser cutting of stents has become the preferred choice. When using standard lasers for ablation and melting processing, issues like excessive burr formation, inconsistent slot widths, severe surface ablation, and uneven strut widths often occur. These problems can be effectively avoided with ultrafast laser cutting, ensuring high-quality, and precise stent production.
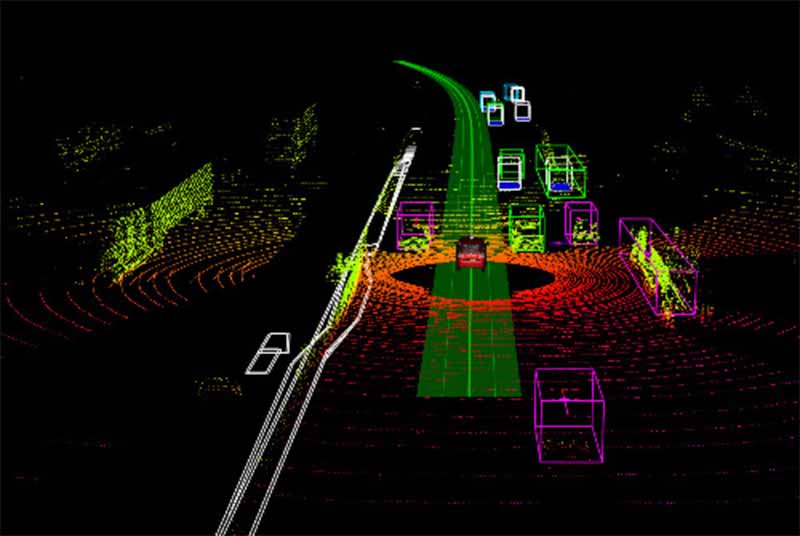
Applications of Ultrafast Lasers in Communications
Ultrafast pulse lasers, with their extremely short pulse duration and high peak power, have become the ideal tool for generating ultra-high-speed optical signals, significantly enhancing communication capacity and speed. These lasers can generate very narrow time pulses, which in the frequency spectrum manifest as extremely wide bandwidths. This property provides optical communication systems with higher frequency resource utilization. By using femtosecond or picosecond pulses, communication systems can transmit more data streams within the same optical fiber, facilitating the efficient implementation of high-frequency multiplexing techniques such as Wavelength Division Multiplexing (WDM) and Time Division Multiplexing (TDM).
Applications of Ultrafast Lasers in Precision Measurement
Ultrafast pulse lasers also play a crucial role in cutting-edge fields like quantum computing and precision measurement. For example, in quantum computing, ultrafast pulse lasers can be used to manipulate quantum bits (qubits), enabling the processing and transmission of quantum information. In precision measurement, ultrafast pulse lasers serve as high-precision time standards, allowing the measurement of extremely small time intervals and lengths. This capability is essential for advancing research and technologies in both quantum systems and high-precision instrumentation.
Ultrashort pulse lasers offer significant advantages in many applications requiring high precision and quality, such as marking, engraving, and cutting. Manufacturers can prioritize this solution due to its extremely short pulse durations and high peak power, which enable extremely high processing accuracy while significantly reducing the heat-affected zone, preventing material deformation or damage. These characteristics make ultrafast lasers suitable for a wide range of materials, including metals, glass, ceramics, plastics, and polymers. They are especially effective when processing thermally sensitive materials or complex structures, demonstrating strong adaptability and stability.
At the same time, their excellent processing quality and ability to handle complex structures are gradually replacing traditional long-pulse lasers in fields such as microelectronics, medical devices, and precision manufacturing, making them the preferred choice for high-end manufacturing.
The Future of Ultra-Fast Laser Applications
Ultra-fast laser technology has undergone significant advancements in recent years, driven by breakthroughs in materials science, photonics, and precision engineering. These innovations have led to the development of lasers capable of generating extremely short pulses, often in the femtosecond or picosecond range, which can be used for a variety of high-precision applications.
As production processes for these lasers have scaled up, costs have decreased dramatically. Mass production of ultra-fast laser equipment has made these once-expensive technologies more accessible, opening up new markets and applications across industries.
In the future, ultrafast laser applications are expected to expand into areas such as smart manufacturing, biomedical technologies, and semiconductor processing, achieving even broader applications. As the technology continues to mature and costs gradually decrease, ultrafast laser technology is likely to become more widely accessible, further driving the growth of the market and its widespread adoption across various industries.